Main Content
Research
Our current themes are:
Ancient ocean analogue ecosystems
Our research seeks to unravel the explicit connections between microenvironmental processes and global-scale transitions in Earth's redox landscape during the Precambrian era. The evolution of oxygenic photosynthesis in cyanobacteria was a milestone on the way to today's oxygen-rich atmosphere. However, much remains unknown about the biogeochemical and ecological interactions surrounding ancient cyanobacteria. These interactions not only influenced atmospheric oxygen levels but also played a crucial role in the fluctuations of greenhouse gases like CO2 and CH4, thereby driving substantial climate changes. Critical in understanding the pattern of Earth’s oxygenation is thus a comprehensive understanding of the regulation of key microbial processes in the ancient carbon cycle. Here, we focus on the regulation of primary production by reduced sulfur, iron and arsenic. These compounds likely facilitated the emergence of the first forms of anoxygenic photosynthesis, exerted crucial control over cyanobacterial oxygenic photosynthesis, and were widespread in productive coastal oceans and microbial mats during the Precambrian.
We therewith work towards a biologically nuanced perspective on Earth’s oxygenation history. How did microbial activity impact the accumulation of oxygen within the context of changes in Earth's geochemistry, the evolutionary onset of different metabolisms, and the evolution of the Earth-Moon system through deep time? To address these questions, we employ two main approaches. First, we study natural analogs of Precambrian ecosystems, such as microbial mats in extreme environments, to constrain the potential role of different types of photosynthesis during major Earth oxygenation events. Second, we simulate conditions in artificial ecosystems within a laboratory setting, enabling us to investigate and understand the dynamics of these processes in depth.
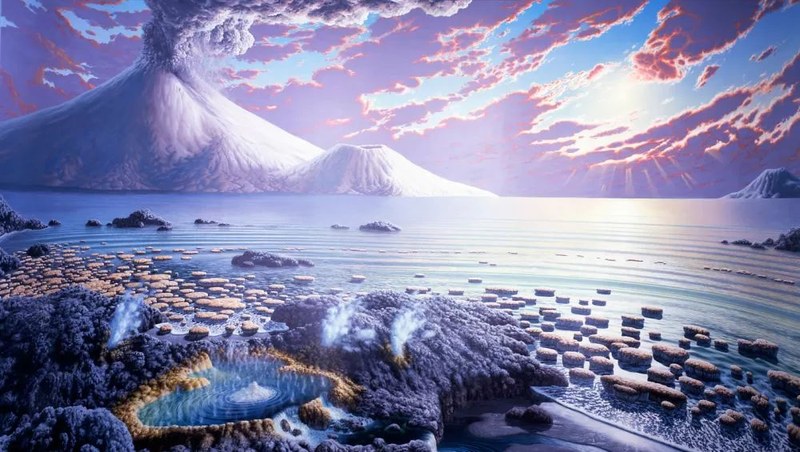
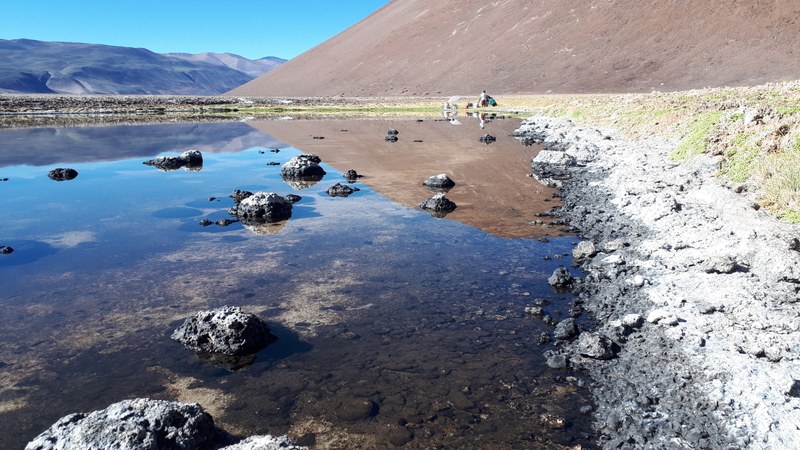
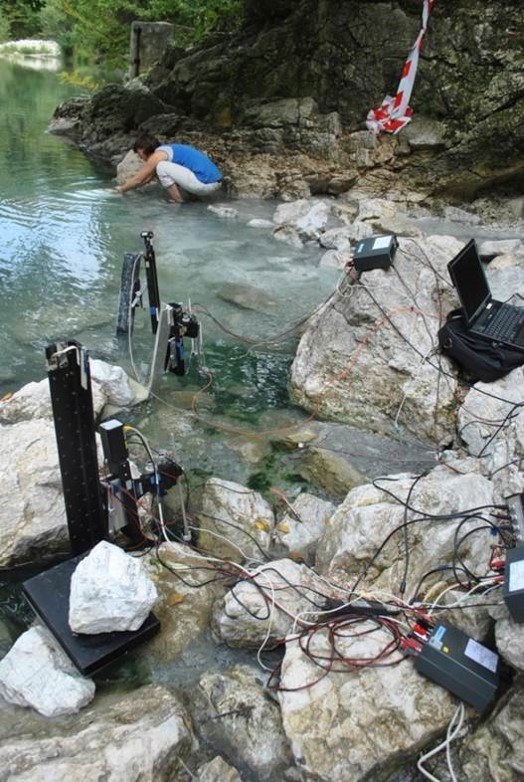
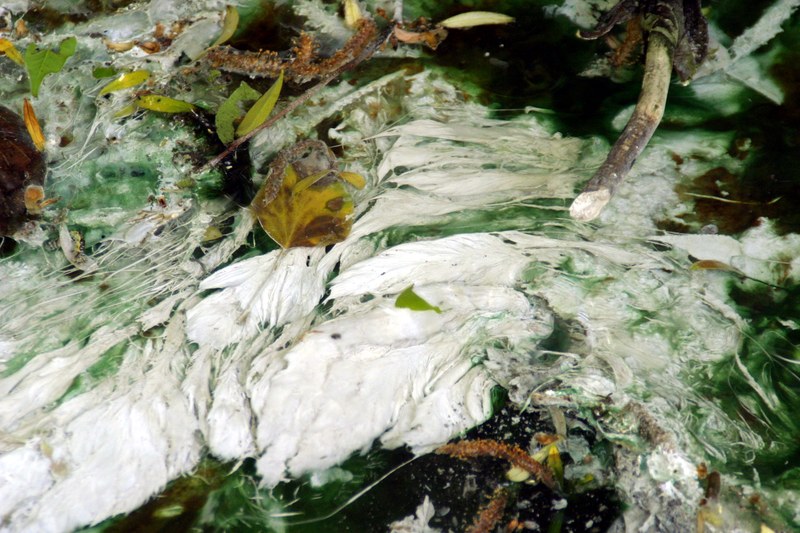
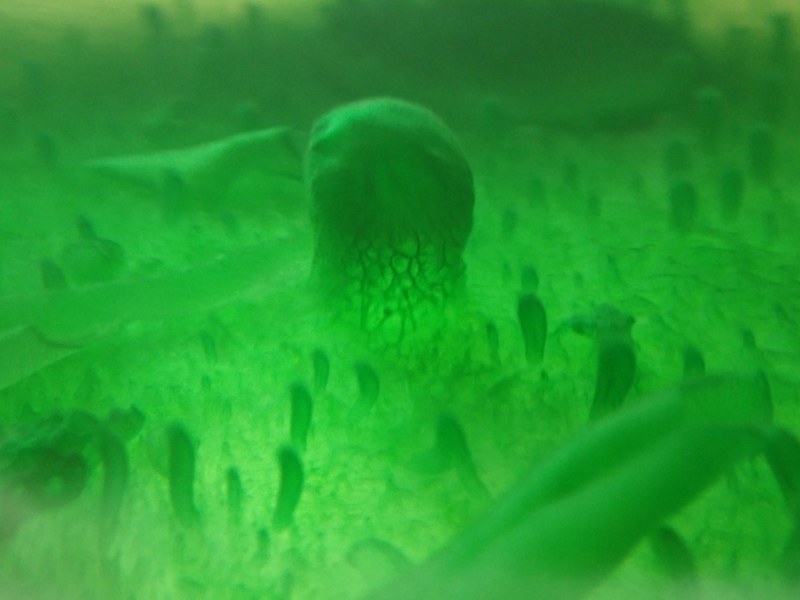
Environmental arsenic cycling
Arsenic contamination is of concern around the world due to its ubiquity and short- and long-term effects on human health. We are therefore in urgent need to limit human exposure to arsenic. Understanding its mobility in the environment is, however, hindered by the complex abiotic and biotic interactions of arsenic with e.g. iron, sulfur and organic matter. We therefore aim to develop novel methods that aid to resolve micro-scale processes in soil and sediment – processes that ultimately dictate arsenic redox cycling and mobility. We are particularly interested in using these tools to characterize light-driven arsenic cycling in benthic systems.
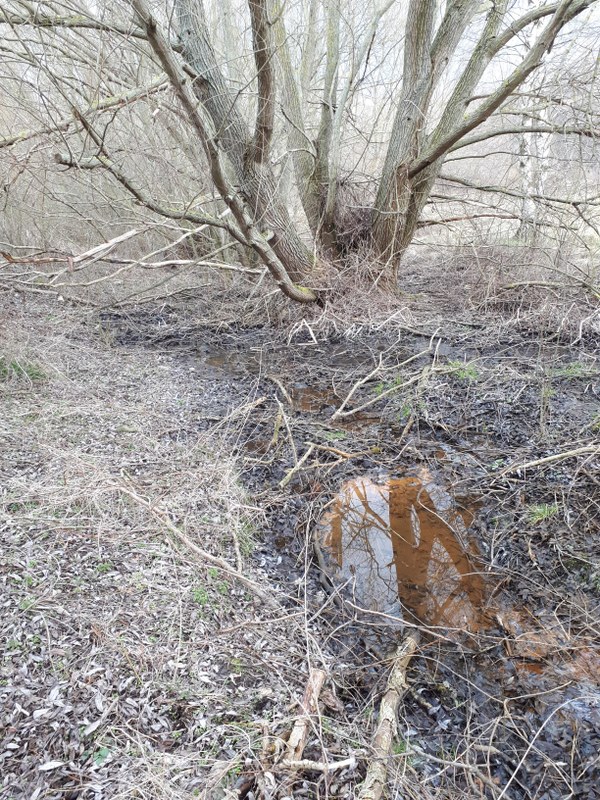
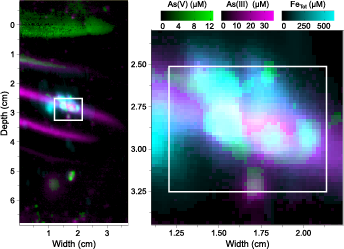
Another important focus is to develop an understanding of arsenic transfer in the larger food web. In high concentrations, arsenic is considered toxic and given its similarity to phosphate, it can easily be incorporated by organisms and may block and interfere with several vital processes. Particularly primary producers, such as cyanobacteria and chlorophytes, incorporate arsenic into carbohydrates and lipids. We aim to (1) characterize the type and origin of organic arsenicals in microbial mats in Andean lagoons and lakes and (2) track their transfer across the food web. Our model organism is Artemia salina, a salt shrimp, feeding on arsenic rich algae, in which we track the fate of organic arsenicals using a combination of classical and image-based mass spectrometry approaches, keeping an eye on its microbiome.
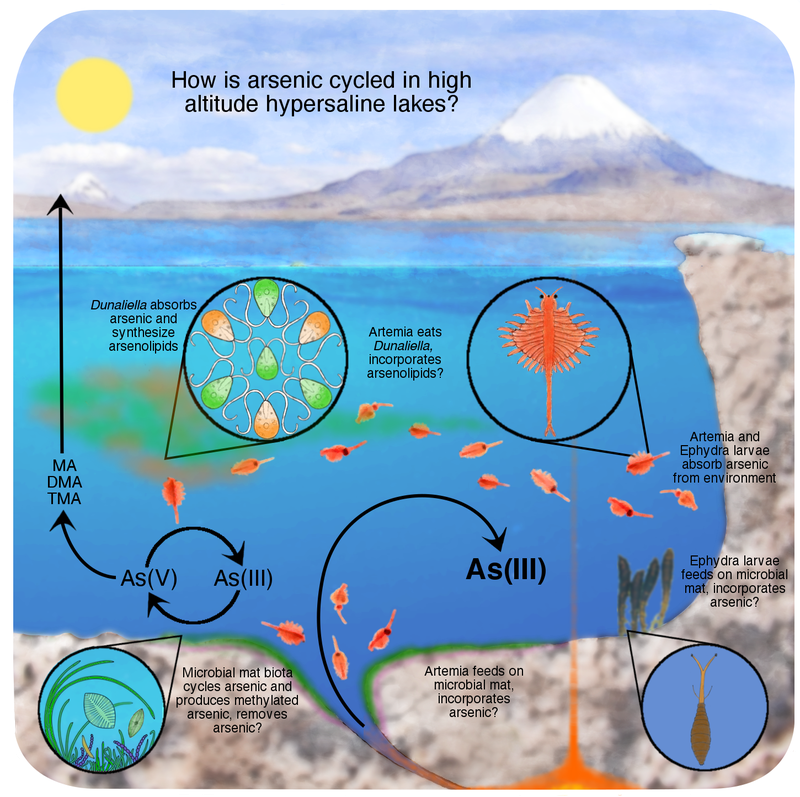
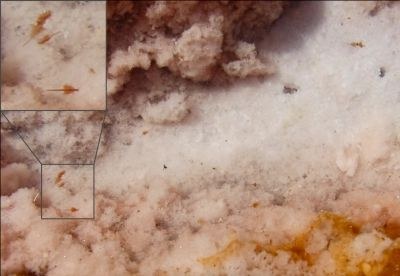
Miraculous diatoms
Diatoms are eukaryotic oxygenic phototrophs and among the most important primary producers globally. Due to their convoluted evolutionary history, diatoms are characterized by a highly unorthodox combination of genes derived from two secondary endosymbionts and from excessive horizontal gene transfer. Even the organization of organelles is exceptional, with, for instance, mitochondria and plastids in very close proximity within the cell. Thus, diatoms may drive processes that are unknown in other Eukaryotes, especially in the absence of light and photosynthesis. We therefore aim to illuminate the metabolic “dark side” of these phototrophs.
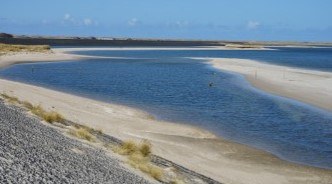
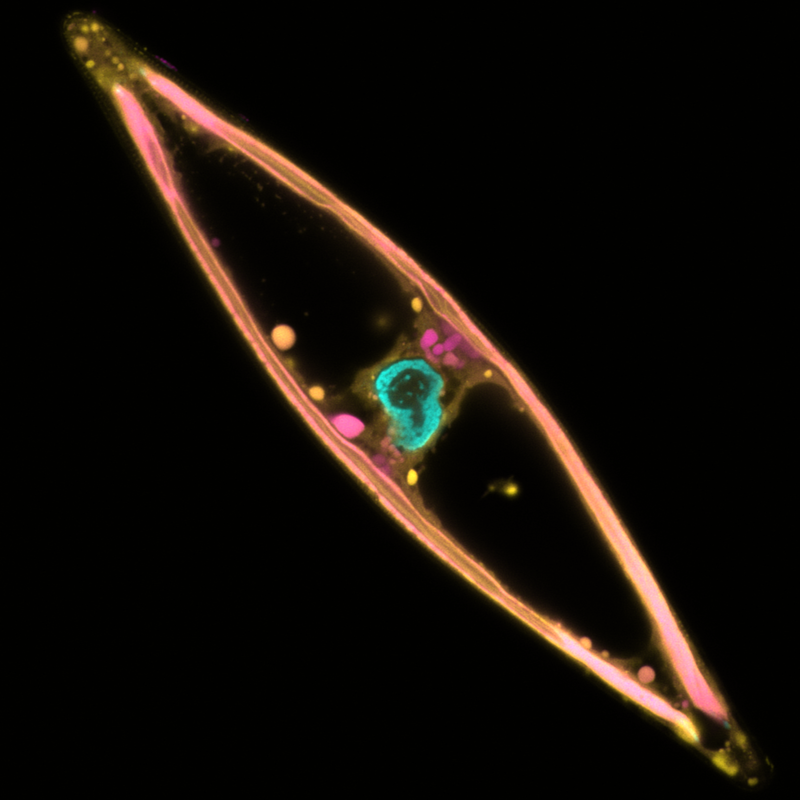
Benthic cycling of reactive oxygen species
Reactive oxygen species (ROS), such as hydrogen peroxide or superoxide, are ubiquitously produced in every aerobic environment and organism. While high concentrations can be cell damaging, ROS were also discovered to be key messenger molecules in cellular pathways and are thus now regarded as “Jekyll and Hyde” molecules. We are particularly interested in the biotic mechanisms of production and scavenging in illuminated sediments and mats. Our favourite study organisms are large benthic foraminifera that thrive in coral reef sediments and substantially shape the sedimentary ROS budget.
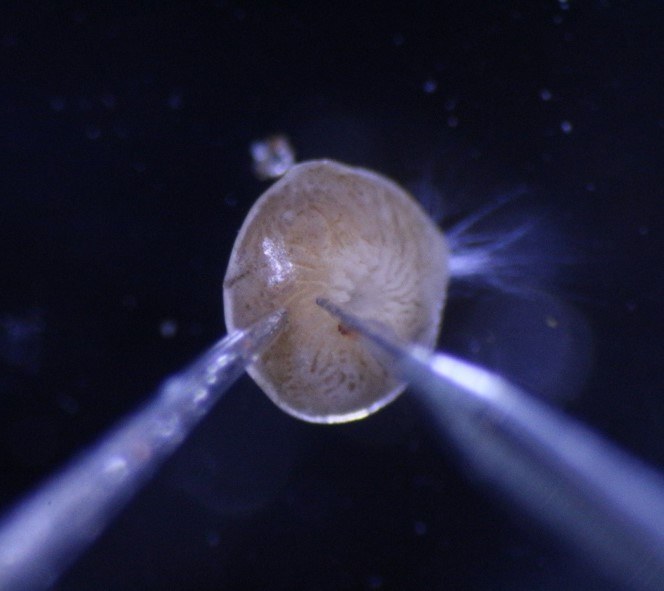