Main Content
Stress responses of the soil bacterium Bacillus subtilis to changing osmolarity
The Gram-positive bacterium Bacillus subtilis lives in the upper layers of the soil. Bacilli can also grow in marine habitats and in association with either plant roots or plant leaves. B. subtilis must therefore adapt to various environmental conditions and variations in nutrient supply. B. subtilis forms highly stress-resistant endospores when the conditions in the environment are unfavourable and the supply of nutrients is scares. These spores can persist for many years in the environment and when the conditions are more favourable they are revived and allow the formation of vegetative B. subtilis cells.
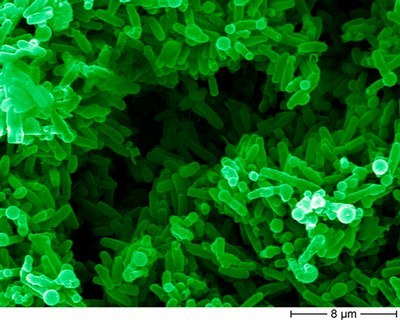
Electron microscopy of the Bacillus subtilis strain JH642. The picture was kindly provided by Christian Arnhold and Dr. K.-H. Rexer; Philipps-University Marburg
Monitoring and adapting to changes in environmental conditions are critical processes that determine the survival of microorganisms and their successful long-term competition for a given habitat. Our group focuses on the physiological and molecular mechanisms by which bacteria respond to changes in the environmental osmolarity and we use the gram-positive soil bacterium Bacillus subtilis and closely related genera as our model systems.
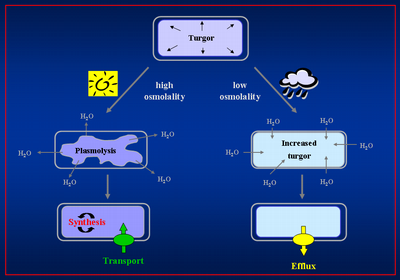
The osmostress response of Bacillus subtilis
All microorganisms must cope with changes in water availability in their milieu since the concentration of solutes within the cell is higher than that in the environment. This will cause an influx of water into the cell and a concomitant build-up of a hydrostatic pressure, the turgor, which rests on the elastic murein sacculus. Turgor has been estimated at 19 x 105 Pa (19 bar) for B. subtilis, a pressure that is approximately ten times higher then that that present in a car tire. Sustaining such a difference on opposite sides of the semipermeable cytoplasmic membrane is essential since a positive turgor is considered as the driving force for cell expansion. Changes in the external osmolarity trigger rapid water fluxes along the osmotic gradient and thus cause either swelling and eventually rupture of the cell in hypotonic environments or plasmolysis and dehydration of the cytoplasm under hypertonic conditions. Microorganisms lack the ability to actively transport water in or out of the cell, and osmotic processes consequently determine their water content. To maintain turgor within physiological acceptable boundaries under changing environmental conditions, they must, therefore, actively manage their intracellular solute pool. To cope with hyperosmotic conditions, microorganisms amass initially large amounts of K+ and in a second step a selected group of organic osmolytes, the so-called compatible solutes, to retain a physiological suitable level of cell water and turgor. They then rapidly expel these compounds through mechanosensitive channels when they are exposed to hypoosmotic circumstances that will otherwise result in a strong influx of water, build up of turgor and eventually rupture of the cell.
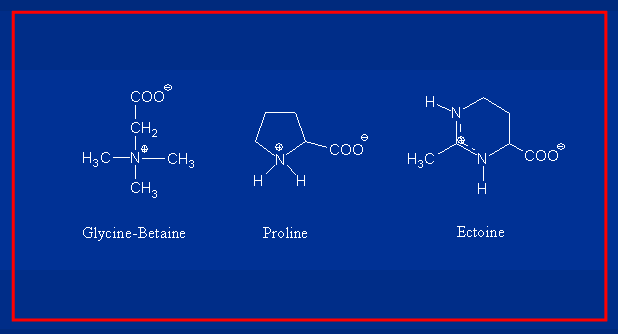
The most important compatible solutes for Bacilli
The intracellular amassing of compatible solutes as an adaptive strategy to high osmolarity environments is evolutionarily well-conserved in Bacteria, Archaea and Eukarya. Important examples of compatible solutes are the amino acid proline, the trimethylammonium compound glycine betaine and the tetrahydropyrimidine ectoine, all of which are accumulated to high intracellular levels by B. subtilis and related Bacillus spp. either through synthesis or uptake from the environment.
The challenge posed by changing environmental osmolarity is vividly illustrated by the common habitat of B. subtilis, the upper layers of the soil. Drought and rain drastically alter the osmotic conditions within this ecosystem and threaten the cell with dehydration or rupture. The initial response of this bacterium to sudden increases in the external osmolarity is the uptake of large quantities of K+ to counteract the immediate outflow of water from the cell. We identified two K+ transport systems (KtrAB; KtrCD) that mediate rapid K+ uptake subsequent to an osmotic up-shock. B. subtilis strains that lack these systems can not resume growth when they face a sudden hyperosmotic challenge and they also exhibit a severe growth defect upon prolonged propagation in hypertonic media. Hence, K+ uptake is required for both the initial as well as for the sustained stress response of B. subtilis to hyperosmotic environments. However, because prolonged high intracellular concentration of K+ are detrimental to many physiological functions (e.g., protein synthesis), B. subtilis replaces in the second step of its osmoadaptation process part of the accumulated K+ with the compatible solute proline through de novo synthesis. We found that B. subtilis possesses an osmoresponsive proline biosynthesis pathway that is different from that used by this bacterium for anabolic purposes. Through this stress responsive synthesis pathway, B. subtilis is able to produce very large quantities (700 mM - 1 M) of proline and can thereby partially offset the detrimental consequences of high salinity and osmolarity. Mutants lacking this osmoregulated proline biosynthetic pathway have a distinct growth disadvantage in hypertonic media.
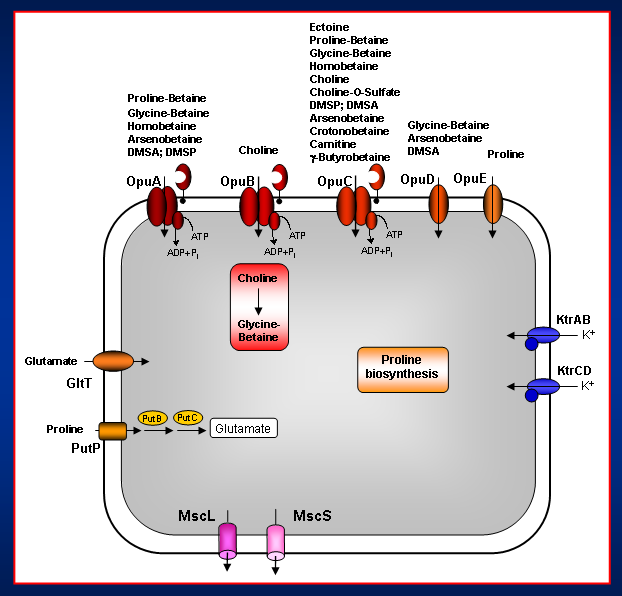
The osmo-stress response systems of Bacillus subtilis
Using 13C-NMR spectroscopy we have studied the types of compatible solutes produced by a wide variety of Bacillus species and closely related genera. We found that may Bacillus species synthesize the tetrahydropyrimidine ectoine instead of proline as their dominant compatible solute. Using molecular approaches, we unraveled the osmoregulated ectoine biosynthetic pathway in B. pasteurii.
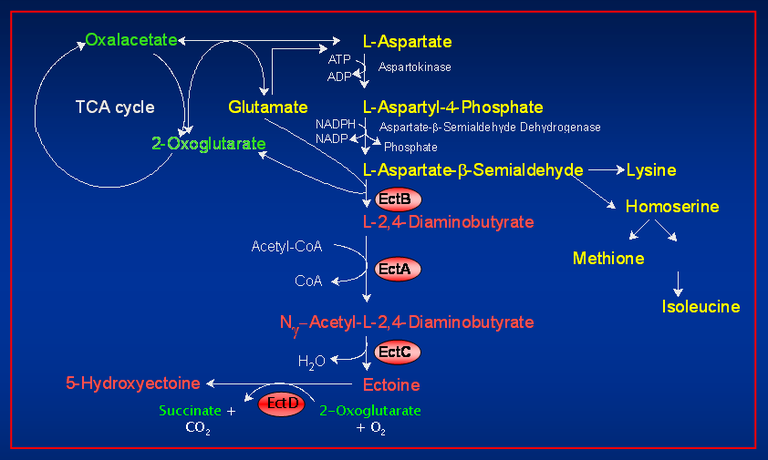
Biosynthetic pathway for the compatible soluts ectoin and hydroxyectoine in Bacilli.
In addition to the de novo synthesis of proline, B. subtilis can also use exogenously provided proline as an osmoprotectant. We identified an osmoregulated, high-affinity transport system, OpuE (Fig. 1) (Opu denotes osmoprotectant uptake) that is responsible for the acquisition of proline for osmoprotective purposes. The opuE gene is expressed from two osmotically stimulated promoters, one of which is controlled by the alternative sigma factor SigB, the master regulator for the large general stress regulon of B. subtilis. B. subtilis can not only use exogenously provided proline for osmostress protection but also as an effective source for carbon and nitrogen. When proline is acquired for nutritional purposes, it is taken up through the PutP transporter and is then degraded to glutamate via the PutB and PutC enzymes.
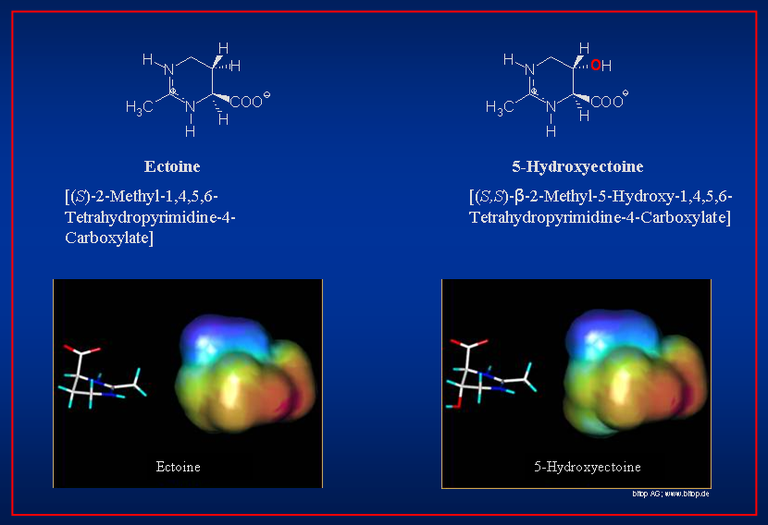
Chemical structures and ab initio calculations of ectoine and hydroxyectoine
In addition to the synthesis of proline for osmostress protection, B. subtilis can acquire a broad spectrum of preformed compatible solutes from the environment through transport processes. These osmoprotectants are synthesized by plants and are thus brought into the habitats of B. subtilis by root exudates and decaying plant tissues. Their availability is likely to vary considerable in the soil and other habitats of B. subtilis, and consequently, effective mechanisms are required for the acquisition via transport from the environment. We found that the uptake of osmoprotectants from exogenous sources involve fife osmoregulated and high-affinity transport systems. Three of these (OpuA, OpuB, OpuC) are multi-component transporters and are members of the ATP-binding cassette (ABC) superfamily and two (OpuD, OpuE) are single-component transporters. Each member of the Opu-family of transporters is osmoregulated at the level of transcription. They differ however in their kinetic properties and substrate specificity.
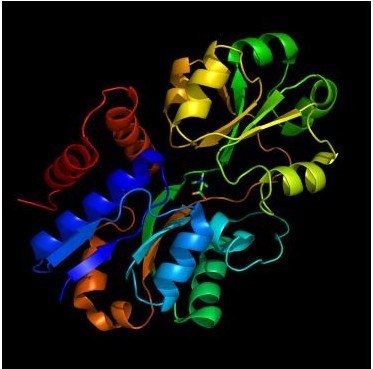
Crystal structure of the glycine betaine binding protein OpuAC from Bacillus subtilis with bound ligand (see Horn et al. J.Mol.Biol. 357:592-606; 2006)
With the exception of proline, the compatible solutes used by B. subtilis are all metabolically inert and they can also not be synthesized de novo by this soil bacterium. However, the potent osmoprotectant glycine betaine can be produced by B. subtilis provided that the precursor choline is present in the growth medium. Choline uptake is mediated by two evolutionarily closely related ABC transporters: OpuC, a transporter with a broad substrate specificity, and the highly substrate-specific transport system OpuB. The choline taken up by the cells is oxidized by the GbsA and GbsB (Gbs denotes glycine betaine synthesis) enzymes to the metabolically inert compatible solute glycine betaine. Both the opuB and gbsAB operons are up-regulated in response to the presence of choline in the growth medium and this genetic control of opuB and gbsAB transcription is mediated through the repressor protein GbsR.
The very same compounds that protect B. subtilis from the detrimental effects of high osmolarity become a threat to its survival when the cells are exposed to hypoosmotic conditions since this will trigger a rapid influx of water into the cell. The cell must therefore rid itself rapidly of ions and organic osmolytes to curb the increase in turgor. B. subtilis accomplishes this via mechanosensitive channels exhibiting different levels of conductances. We identified two such channels: MscL and MscS. B. subtilis mutants lacking the MscL and MscS proteins can not survive severe osmotic down-shocks.
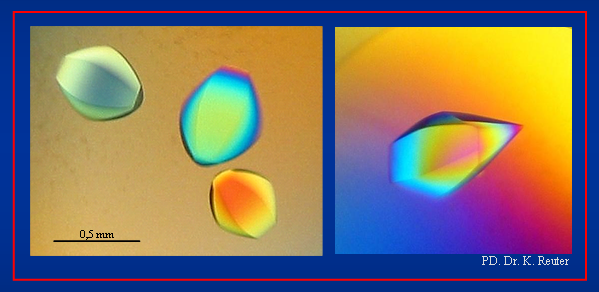
Crystals of the ectoine hydroxylase EctD from Salibacillus salexigens (Picture provided by Dr. K. Reuter; University of Marburg)
The uptake and synthesis of osmoprotectants confers a considerable degree of osmotolerance to B. subtilis. The pathways for the production and transport of compatible solutes will serve in future studies as useful model systems to unravel the mechanisms of osmosensing in this soil bacterium and to further study the cellular adaptation response of B. subtilis to a changing environment.
We are also conducting structual analysis of compatible solute binding proteins from various microorganisms in collaboration with the laboratories of Prof. W. Welte (University of Konstanz) and Prof. L. Schmitt (University of Duesseldorf). One of these binding proteins that we are analysing by structural and mutational approaches is the glycine betaine binding proteine OpuAC from B. subtilis. In addition, in collaboratin with Dr. K. Reuter (University of Marburg) we are conducting structural analysis of enzyms required for the synthesis of compatible solutes such as the ectoine-hydroxylase EctD from the moderate halophile Salibacillus salexigens.