Main Content
Research goals
The continuing trend to miniaturization of devices in modern technology faces fundamental physical limits of applied materials. The search for novel structures with new functionalities has brought atomically thin two-dimensional (2D) nanomaterials including graphene, transition metal dichalcogenides (TMDs) and their an der Waals heterostructures into the focus of current research. They represent a new class of materials that are characterized by a wide range of exceptional optical, electronic, mechanical, chemical, and thermal properties suggesting technological application in next-generation flexible and transparent nanoelectronic devices.
Monolayers of TMDs with molybendum and tungsten disulphide and diselenide (MoS2, MoSe2, WS2,WSe2) as their most prominent representatives show a direct bandgap and are characterized by a strong exciton physics including bright as well as momentum- and spin-dark exciton states. The intriguing exciton physics becomes even richer, when atomically thin materials are vertically stacked to form van der Waals heterostructures. The strong Coulomb interaction gives rise to spatially separated interlayer excitons, where the involved electrons and holes are localized in different layers. Another fascinating aspect of theses heterostructures is the appearance of spatially periodic moiré potentials due to the lattice mismatch of adjacent layers. Adding a twist angle one can externally tune these moiré potentials, which presents a unique opportunity to design tailored quantum interfaces and engineer their optoelectronic landscape. One important consequence is that excitons can be trapped in the moiré potential, which can give rise to new exotic quantum phenomena, such as topological exciton insulator states and superconductivity.

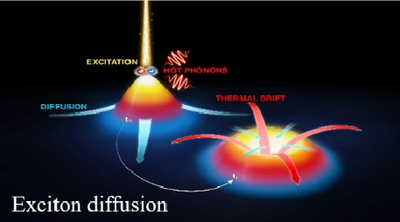
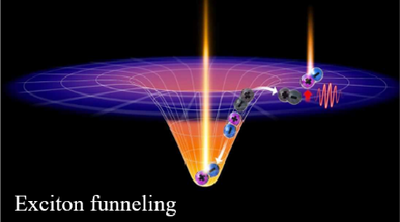
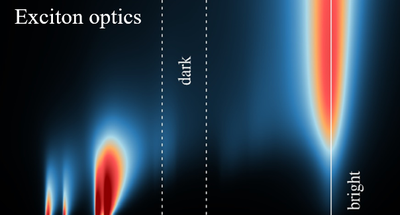
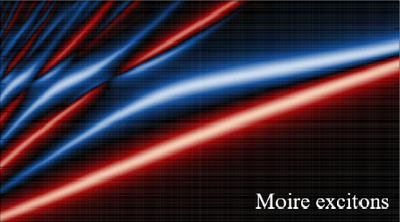
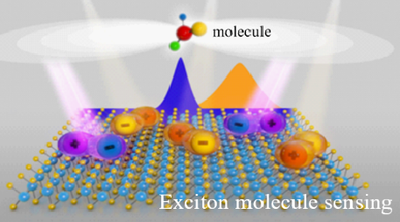
Our goal is to provide microscopic insights into the fascinating world of excitons in this class of materials. Based on the density matrix formalism, we develop material-specific and realistic models to describe many-particle processes behind experimentally accessible phenomena in photoluminescence, absorption, pump-probe, time-resolved ARPES, diffusion and photocurrent measurements. Our focus lies in particular on the ultrafast quantum dynamics in TMD monolayers as well as homo and heterobilayers. Using fully quantum mechanical models, we microscopically track the temporally, spectrally and spatially resolved formation, thermalization and decay of excitons.
We are interested in particular in the following topics:
- Exciton Optics including indirect signatures of dark exciton states
- Exciton Dynamics including formation, thermalization and decay mechanisms of excitons
- Exciton Diffusion and Funneling in density, temperature and strain gradients
- Moire Excitons including their signatures in optics, dynamics and transport experiments
- Exciton Polaritons in strong coupling regimes including cavities or plasmons
- Exciton-Based Sensing concepts for molecules, strain, and light
- Exciton-Electron interactions in monolayered TMDs and TMD-based heterostructures