28.08.2020 New Funding for Molecular Simulations at Steinbuch Centre for Computing and Tomographic Studies at Karlsruhe Nano Micro Facility, Karlsruhe Institute of Technology (KIT)
New funding has been achieved for Molecular Simulations and Electron Tomography for upcoming studies in our group.
- Molecular simulations at Steinbuch Centre for Computing (SCC) supported by >15.000.000 core-hours for the next two years
- FIB-SEM tomography and electron tomography at Karlsruhe Nano Micro Facility (KNMF) supported through a long-term user proposal for the next two years
Molecular simulations at Steinbuch Centre for Computing (SCC) supported by >15.000.000 core-hours for the next two years
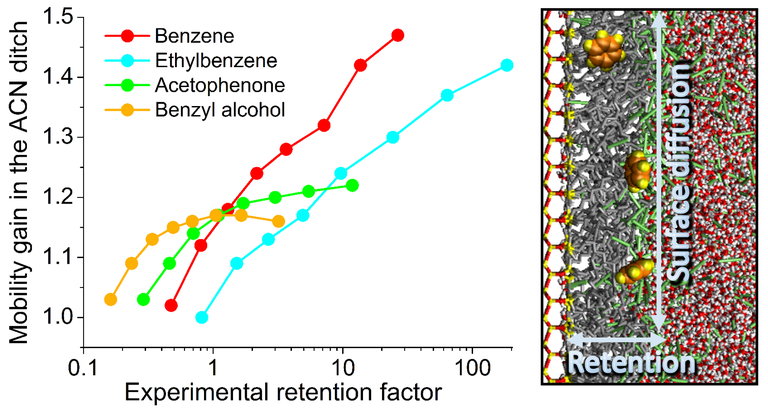
A series of molecular dynamics (MD) simulations studies by our group provided a molecular-level picture of surface diffusion in reversed-phase liquid chromatography (RPLC) at C18- and C8-modified silica surfaces equilibrated with water–acetonitrile (W‒ACN) mobile phases. Key to surface diffusion is the presence of the ACN ditch, an ACN-rich border layer between bonded phase and mobile phase that results from accumulation of ACN molecules around the alkyl chain ends during equilibration of the stationary phase with the mobile phase as a consequence of the hydrophobic effect. The ACN-rich environment facilitates the diffusive mobility of bonded-phase groups, ACN, and analyte molecules, which explains why analyte molecules in contact with bonded-phase groups (i.e., retained by the stationary phase) can diffuse faster along the stationary-phase surface than in the bulk mobile phase. The lateral mobility gain from surface diffusion in the ditch depends on the ACN excess, which decreases with increasing ACN content of the mobile phase as does the retention factor of an analyte. The lateral mobility gain from surface diffusion also depends on stationary-phase properties, namely length and ligand density of the alkyl chains, which determine position, width, and local solvent composition of the ACN ditch as well as the mobility of the bonded-phase groups there. So far, we have elucidated the origin of surface diffusion in RPLC, investigated the relation between surface diffusion and analyte retention, and determined the influence of principal analyte and stationary-phase properties on the lateral mobility gain from surface diffusion. All of these studies were conducted in a planar slit-pore model with W‒ACN mobile phases and analyte concentrations in the linear range of the adsorption isotherm as used in analytical chromatography (i.e., for separation).
In future projects, we want to expand our knowledge of mass transfer mechanisms in RPLC to i) cylindrical pores as most realistic approximation to the mesopore geometry found in chromatographic beds and ii) analyte concentrations and mobile phases used in preparative chromatography (i.e., for purification). The first point, moving from planar slit-pore models to cylindrical pore models, is a major undertaking that involves significant computational effort as well as time-consuming preliminary simulations to determine the exact number of solvent molecules in the simulation box necessary to replicate the targeted experimental conditions. That is why molecular simulations of chromatographic systems in cylindrical pores have rarely been attempted so far. The second point, modeling nonlinear adsorption through molecular simulations has never been attempted before. We undertake this project because we can rely on our experience with MD simulations of chromatographic systems as well as on our background in experimental chromatography. By systematically increasing the analyte concentration up to the range of column overloading in preparative chromatography, we plan to recover the adsorption isotherms of selected analytes and provide a molecular-level picture of the chromatographic interface at different critical points of the adsorption isotherm. Studies of nonlinear adsorption will be carried out for W‒ACN as well as W‒MeOH mobile phases, as experiments have shown a decided influence of the organic solvent on the isotherm.
Further reading:
J. Rybka, A. Höltzel, U. Tallarek, Surface diffusion of aromatic hydrocarbon analytes in reversed-phase liquid chromatography. J. Phys. Chem. C 2017, 121, 17907–17920.
J. Rybka, A. Höltzel, A. Steinhoff, U. Tallarek, Molecular dynamics study of the relation between analyte retention and surface diffusion in reversed-phase liquid chromatography. J. Phys. Chem. C 2019, 123, 3672–3681.
J. Rybka, A. Höltzel, N. Trebel, U. Tallarek, Stationary-phase contributions to surface diffusion in reversed-phase liquid chromatography: Chain length versus ligand density. J. Phys. Chem. C 2019, 123, 21617–21628.
FIB-SEM tomography and electron tomography at Karlsruhe Nano Micro Facility (KNMF) supported through a long-term user proposal for the next two years
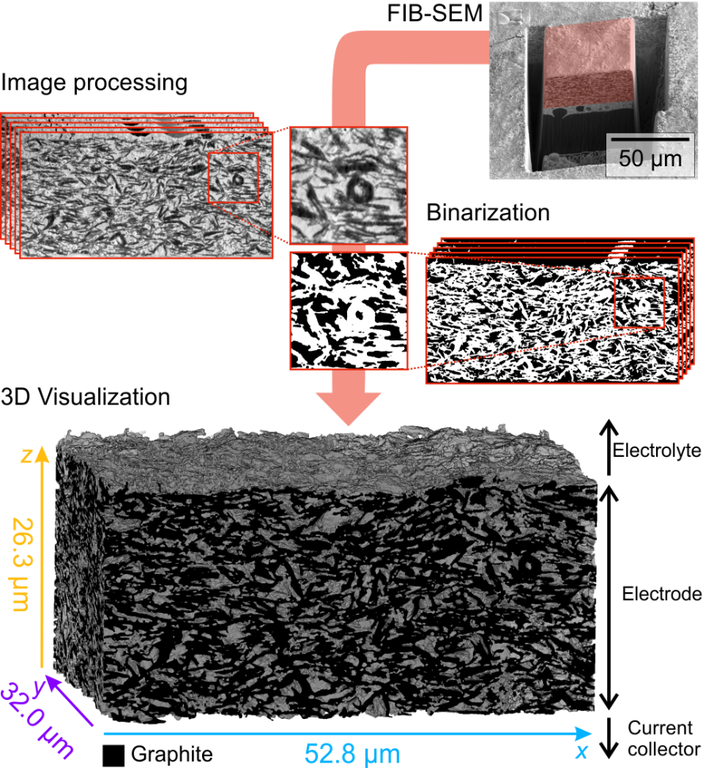
Porous materials have numerous important applications, e.g., in battery electrodes, heterogeneous catalysis, or liquid chromatography. For example, in lithium-ion batteries, the morphology of the (μm-sized) macropores between active particles determines lithium ion transport pathways and, thus, the ion transport resistance of a battery. In high-performance liquid chromatography (HPLC) columns or microreactors, the morphology of the porous particles used as packing materials impacts the process performance via the diffusive mass transfer resistance engendered by the intraparticle pore network of (nm-sized) mesopores. Accurate reconstruction and characterization of porous materials enables the investigation of morphology–transport relationships and to rationally design pore structures and thereby address relevant transport properties in a given application. For example, the morphology of battery electrodes strongly depends on the preparation protocol. Here, we focus on the influence of solid electrolyte and polymeric binder on lithium ion transport in the electrolyte phase of all-solid-state batteries. For this purpose, the electrode morphology is reconstructed using tomographic data obtained by focused ion-beam scanning electron microscopy (FIB-SEM). We intend to quantify rate capability limitations by a combination of electrochemical measurements, morphological analysis, and direct, i.e., pore scale 3D simulations of the electrochemical processes in the obtained reconstructions serving as geometrical models.
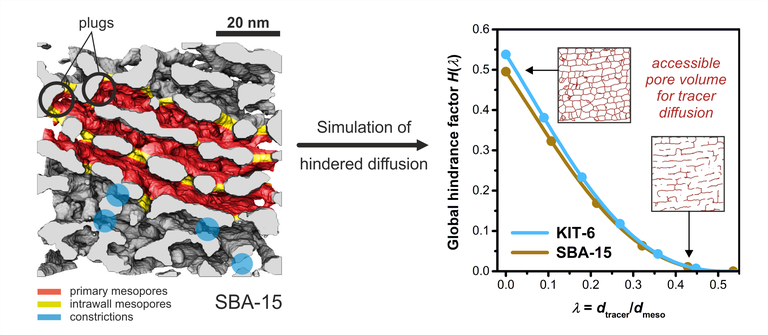
In another application, the quantification of molecular transport through porous particles helps to predict the performance of functionalized mesoporous silica employed as packing material for HPLC columns and continuous-flow microreactors. The effective intraparticle diffusion coefficient reflects all parameters that affect molecular transport in the mesopore volume of a particle. In our approach, diffusion through the mesopore space of the particles is simulated by a random-walk particle tracking technique to determine the diffusion coefficient in dependence of the tracer size. As geometrical models for pore-scale simulations with this approach we use reconstructions of the materials obtained by scanning transmission electron microscopy (STEM) tomography. Simulations are complemented by a morphological study of the mesopore space, with a focus on its topology and geometry, using chord length distributions (CLDs) and medial-axis analysis (MAA). Complementary insights (reconstruction and simulation) provide a complete view on key morphology–transport relationships at the mesopore scale, which are essential to the performance of these materials. Most promising materials in HPLC and catalysis under confinement are silica materials with highly controlled mesopore space properties, e.g., a narrow pore size distribution or ideal cylindrical pore shape. Recently, the reconstruction–simulation approach has been applied to silica particles for use in size-exclusion chromatography. In upcoming studies, we will investigate self-similarity of mesopore networks in materials with different particle sizes and mesopore diameters by the reconstruction–simulation approach. In another study, silica particles with different mean mesopore sizes are investigated in continuous flow microreactors used for catalysis under confinement (ring-closing metathesis of olefins). Quantitative relationships between particle morphology and effective hindered diffusion may lead to a boost in confinement-based selectivity through tailored particle design.
Further reading:
D. Hlushkou, A.E. Reising, N. Kaiser, S. Spannenberger, S. Schlabach, Y. Kato, B. Roling, U. Tallarek, The influence of void space on ion transport in a composite cathode for all-solid-state batteries. J. Power Sources 2018, 396, 363–370.
J. Hochstrasser, A. Svidrytski, A. Höltzel, T. Priamushko, F. Kleitz, W. Wang, C. Kübel, U. Tallarek, Morphology–transport relationships for SBA-15 and KIT-6 ordered mesoporous silicas. Phys. Chem. Chem. Phys. 2020, 22, 11314–11326.
F. Ziegler, J. Teske, I. Elser, M. Dyballa, W. Frey, H. Kraus, N. Hansen, J. Rybka, U. Tallarek, M.R. Buchmeiser, Olefin metathesis in confined geometries: A biomimetic approach toward selective macrocyclization. J. Am. Chem. Soc. 2019, 141, 19014–19022.